
Open Letters
THE ORION PARTY
The Prometheus League
- Humanity Needs A World Government PDF
- Cosmos Theology Essay PDF
- Cosmos Theology Booklet PDF
- Europe Destiny Essays PDF
- Historical Parallels PDF
- Christianity Examined PDF
News Blogs
Euvolution
- Home Page
- Pierre Teilhard De Chardin
- Library of Eugenics
- Genetic Revolution News
- Science
- Philosophy
- Politics
- Nationalism
- Cosmic Heaven
- Eugenics
- Future Art Gallery
- NeoEugenics
- Contact Us
- About the Website
- Site Map
Transhumanism News
Partners




Thalamic Inhibition in the Evolution of Human Intelligence: Evolutionary Pressure for Cortical Inhibition
K. K. Glendenning
Florida State University
One characteristic of a civilized society is the ability and propensity of
its members to refrain from acting in a way that disrupts the harmony of the
group. While this is not intelligence as measured by IQ, it is a form of
social intelligence that will aid those having it in their ability to
propagate. This characteristic is not unique to human societies but rather is
true of most social species.
This report deals with one aspect of brain chemistry that may underlie
this social intelligence, defined as the ability of a species to inhibit its
thoughts as well as its behaviors. Selective pressure has unrelenting
consequences on the mechanisms of brain function, and perhaps the first
mechanism to be affected is the chemistry of the brain. Changes in brain
chemistry and function can be explored by an investigation of species selected
to represent the phylogenetic sequence leading to mankind. In an examination
of nine species selected on this basis, we have found that one consequence of
selective pressure is an increase in the potential for cortical inhibition. We
suggest this may be a major component of the evolution of human intelligence.
Key words: intelligence, thalamus, inhibition, comparative psychology,
evolution, mammals, receptor binding, GABA, muscimol binding, amniotic
vertebrates.
By treating relative brain size as a measure of intelligence, it is
possible to develop a coherent story about the probable history of
intelligence as a biological phenomenon. Like other biological processes,
intelligence must have evolved under the influence of natural selection. That
is, a well known prime result of evolutionary pressure has been the selection
for human intelligence.
The intelligence of humans has been shown to be correlated with an
increase in size of the neocortex (eg., Ariens Kappers 1929; Diamond and Hall
1969). In this investigation we have extended this axiom one step further. Not
only do humans have a larger cortex but we have found that humans also come
equipped with an increased capability of inhibiting this expanded cortex.
To begin this investigation we began with the assumption that a look at
our ancestral heritage gives us insight as to what the future may bring. In
turn, an even broader spectrum of evolutionary analysis can lead to a similar
insight and perhaps predictions about the path along which natural selection
is taking us. That is, what lies ahead in the evolution of mankind is only a
subpart of what has been happening to primates as they are a subpart of what
has been happening to mammals and so on. By an examination of diverse species
chosen to represent a phylogenetic series leading to man, this report shows
one step in the consequences of selective pressure on the mechanisms of brain
function. Our conclusion is that this step, an increase in the potential for
inhibition of the cortex, is probably a major component of the evolution of
human intelligence.
To draw this conclusion we must first examine the results of selective
pressure on brain development. Secondary and tertiary synaptic levels in brain
structure are relatively immune to changes in a species ecological niche.
Natural selection first acts upon those parts of the brain most easily changed
so they can revert with changing circumstances. For example, a species driven
into a nocturnal habitat is subject to adaptive pressure on its visual system
to adapt. The first noticeable gross anatomical changes are the receptors in
the eyeball (Polyak 1957). Prior to these anatomical changes are changes in
the chemistry of the brain. These are the most easily changed and therefore
the first to be effected by selective pressure.
Yet the chemistry of the brain demonstrates a remarkable robustness
throughout phylogeny. In this study we have looked at the constancy of an
inhibitory neurotransmitter in the brain over a wide range of species.
Particular attention has been paid to a part of the brain, the dorsal
thalamus, through which all sensory information is channeled before it reaches
the cortex.
With the advent of modern neurochemical analysis, the notion of the dorsal
thalamus as a `relay' of sensory information to the cortex has given way to
the notion that it is a `gateway' to cortex. In this conception, neural
activity directed to the forebrain is actively modulated by a variety of
inhibitory feedback loops.
An evolutionary dimension to the intrinsic modulation by the dorsal
thalamus can be traced to Ramon y Cajal (1909, 1966) who proposed that the
number of interneurons in the dorsal thalamus increased during the course of
the evolution of mammals. In man, then, our greater intellectual capacity may
not be just a function of our cortex but also of our dorsal thalamus. Further,
it seems that the dorsal thalamus gates the information getting to the cortex
by inhibiting much of it. The idea that the interneurons in the dorsal
thalamus are usually inhibitory has been demonstrated in studies showing that
most neurons are g-aminodecarboxylase (GAD)-immunoreactive and that more
GAD-immunoreactive interneurons are present in species `higher' in an
evolutionary sequence leading to humans (Penny et al.1983,1984).
In the present study, the idea of an increasing amount of g-aminobutyric
acid-A (GABA-A) inhibition over the Anthropoid ancestral lineage was
investigated using seven mammals and two non-mammals selected to yield at
least 5 differing grades or levels of recency of last common ancestor with
Anthropoids. Any commonality among these would be relevant to the prototype of
our common ancestor. Moreover, any change in pattern could be used to predict
the direction these selection factors will have on the evolution of brain
chemistry.
These species, each subjected to exactly the same receptor-binding
procedure, were compared for the relative distribution of muscimol bound
GABA-A receptors among the four subdivisions of the diencephalon.
The results support the view that the presence of g-aminobutyric acid and
its role in inhibiting neurons in the dorsal thalamus is probably a
fundamental characteristic of amniotes changing only in amount during the
later evolution of mammals. This has implications for an increasing role of
inhibitory synapses in mammals, in primates, and perhaps most importantly to
us, in humans.
Materials and Methods
The labeling of high-affinity GABA-A receptors was accomplished by
conventional receptor binding methods.(eg, Glendenning and Baker 1988, Cole et
al.,1984, Nauta and Karten 1970, Penney et al., 1981). Tritiated muscimol was
used as the labeling ligand and GABA itself was used as the displacing agent
for matched sections of control tissue (e.g., Snodgrass 1978)
For controls, myelin-stained material and binding of two other receptors
(serotonin and muscarinic acetylcholine (ACh) was also performed to be sure
that the pattern of muscimol binding among the diencephalic subdivisions was
not merely the secondary result of differences in cell packing, neuropil
density or total available membrane.
Sample Species
In all, 26 individuals from the 9 species were examined. Species were
selected mainly on paleontological, taxonomic, and comparative anatomical
grounds for their sequential recency of last common ancestry with Anthropoids
(Fig. 1). This selection allowed the sample to contain mammals with at least 4
grades of successive kinship with Anthropoids: preplacental, two preprimate,
and primate levels (e.g., Cronin and Sarich 1980, Dene et al., 1980, Luckett
1980, McKenna 1975, Szalay and Drawhorn 1980).
Therefore, the seven species of mammals which could be arranged on the
basis of the remoteness of their last common ancestor with Anthropoids
included Monodelphis and Didelphis opossums (Monodelphis domesticus, Didelphis
virginiana), armadillo (Dasypus novemcinctus), rat (Rattus rattus), tree shrew
(Tupaia glis), bushbaby (Galago senegalensis), and lemur (Lemur fulvus; eg.,
Nudo and Masterton 1988).
To these seven mammalian species, two non-mammalian amniotes with
unarguably remote common ancestry with Anthropoids were added to the sample: a
reptile (Iguana iguana) and a bird (pigeon, Columba livia). Together, the
total of nine species in the sample could be used to deduce at least 5
phylogenetic grades (numbered 0 through 4 in Fig. 1).
Measurement of Receptor Binding
Because of the wide variation in the dissociation constant (KD) for
muscimol-binding, we performed standard statistical analyses of variance of
the dissociation constants in 71 reports published over a 12 year period.
Separate analyses, using `laboratory of origin', `tissue procedure', and
`species' as single factors showed that it was `laboratory' alone which was
the significant factor in the variation of KD values (p < 0.0001). The other
two factors, `tissue-procedure' and `species' singly or with interactions
yielded no significant variation (tissue-procedure, p > 0.7; species, p > 0.8;
tissue-procedure x species, p > 0.8).
Therefore, we chose to standardize the procedure to be certain that the
comparison of muscimol binding among the present species would not vary as a
result of even minor variations. Consequently, the tissues from all 26
individuals in the 9 species were treated identically including exposure to
exactly the same concentration of ligand.
The in vitro receptor-binding technique followed the protocol of
Kuhar(1985) and his colleagues (Nauta and Karten 1970, Zarbin et al., 1981 and
see Beaumont et al., 1978, Glendenning and Baker 1988, Shaw et al.,1984). For
the control binding of serotonin receptors with serotonin and muscarinic ACh
receptors with quinuclidinyl benzilate (QNB), the procedures of Pazos and
Palacios (1985) and Yamamura and Snyder (1974), respectively, were followed.
The individuals were routinely euthanized following the guidelines of the AVMA
Panel on Euthanasia (1993).
Microdensitometry of the Tritium-sensitive Film
For each procedure, the sections were exposed to tritium-sensitive film
along with autoradiographic tritium microscales which were placed with the
sections in the film-cassette (Geary et al.,1985). These microscales allowed
the optical density of the autoradiographic images produced by the bound
ligand to be converted into relative densities.
Microdensitometric analysis was accomplished using a microcomputer imaging
system (Imaging Research, Inc.). Following calibration, a `total' binding
image was digitized and then overlaid with the `non-specific' binding image.
The `specific' binding image
was the difference between them. The tissue for analysis, i.e., dorsal
thalamus, epithalamus, etc., was outlined on the `specific' binding image of
the thalamus and average relative optical densities were generated for each
subdivision. The values shown in the tables and graphs are the averages of at
least twelve photo-density measurements of each subdivision, six from each
side of the brain.
Results
There are three chief results: 1) inhibitory receptors as shown by
muscimol binding was far denser in the dorsal thalamus than in any other of
the four major subdivisions of the thalamus for each of the 9 species; 2) the
pattern of muscimol binding across the four thalamic subdivisions was similar
regardless of the species' taxonomic class, subclass, order, or family; and 3)
only the dorsal thalamus in the placental mammals suggested a systematic
increase in muscimol binding over geological time. Since it is also known that
phylogeny brings an increase in the development of cortex, the systematic
increase in inhibitory receptors in the dorsal thalamus of placental mammals
is correlated with the increase in the development of the cortex of placental
mammals.
Muscimol Binding
In each of the seven mammals, a broad band of high-density binding was
confined to dorsal thalamus.
The microdensitometric measurements of relative muscimol binding in each
of the four subdivisions of the diencephalon is tabulated in Table I. It can
be seen that the density of binding in dorsal thalamus always exceeded that in
the other diencephalic subdivisions. It should be noted that there was also a
relatively high density of bound muscimol in the dorsal thalamus of the
non-mammals, although it was less dense than in the mammals (compare scaling
of vertical axes in Fig. 2). Table I also shows that there was more bound
muscimol in the entire diencephalon of the mammals than in that of the
non-mammals.
The profiles of the relative density of muscimol binding across the 4
subdivisions, with dorsal thalamus arbitrarily standardized to 100%, are
illustrated in Figure 3. It can be seen that the density of muscimol binding
in the dorsal thalamus (the second bar of each graph) is far higher than the
other three subdivisions of the diencephalon while binding in the hypothalamus
is higher than in either epithalamus or ventral thalamus.
Although there was variation in binding among the several species of
mammals, the relative density among subdivisions of the diencephalon was
similar within each species: a dorsal to ventral, low-high-low-high profile
was obtained regardless of species. Therefore, this generalized profile of
bound muscimol across the diencephalic subdivisions may be a common
characteristic of amniotic vertebrates, whether mammalian or not.
It should be noted that in order to produce comparable profiles of
relative binding density among the species, the scale of optical density
differs among the species (compare entries in Table I). This scaling, showing
relative optical density rather than absolute numbers of receptors, allows
comparison among the species despite possibly differing dissociation constants
for muscimol.
Comparison of Profiles of Muscimol Binding with Other Ligands
Before pursuing some possible factors leading to the variation in levels
of muscimol binding, it is noteworthy that two other receptor binding ligands
did not show the same general pattern as that of bound muscimol.
That is, while the dorsal thalamus is heavily bound with muscimol, it is
relatively free of 5-HT binding. Other data leading to the same conclusion
were found in the binding of the other control ligand: QNB binding of mACh
receptors also does not parallel the profile of muscimol binding. Taken
together these two controls make it unlikely that the muscimol profile across
the thalamus is based solely on the differences among the subdivisions in cell
or neuropil density. Therefore, the heavy muscimol binding in the dorsal
thalamus probably is not the secondary result of differences in cell or
neuropil density nor in total available membrane.
Taxonomic Differences in Muscimol Binding
Returning to the muscimol binding results themselves, Figure 2 allows
comparison of the relative amount of bound muscimol in the dorsal thalamus and
in the entire diencephalon, for non-mammalian vs. mammalian species and for
marsupial vs. placental mammals. In
the top graphs it can be seen that a greater density of muscimol binding
was found in the mammals than in the non-mammals in dorsal thalamus (F61, p <
.04).
In the bottom graphs in Figure 2, it can be seen that the marsupials
(Monodelphis and Didelphis opossum) had relatively more muscimol binding than
the placental mammals and this statistically reliable difference appeared both
in the dorsal thalamus alone (F61,p < .04) and in the entire diencephalon
(F61, p < .04).
Possible Phylogenetic Trends in Muscimol Binding
Because reliable mammal/non-mammal differences and reliable
marsupial/placental differences were found in the relative density of muscimol
binding in dorsal thalamus, we were encouraged to analyze the possibility of a
phylogenetic trend along the Anthropoid ancestral lineage. Figure 4 shows the
amount of muscimol binding among the species when they are arranged on the
basis of their nearness of kinship, or recency of last common ancestor, with
Anthropoids and thus with humans (i.e., see Fig. 1).
It can be seen that for the entire group of species, no reliable trend
appears (see dotted line in Fig. 4, left). Nor does a trend appear when the
two non-mammals are omitted and only the seven mammals included (see dashed
line in Fig. 4, left). It is only when the two marsupials as well as the two
non-mammals are omitted (leaving the 5 placental mammals alone) that a
statistically reliable trend in muscimol binding appears in dorsal thalamus
(see solid line in Fig. 4, left). Therefore, despite the small sample size of
placental species (n = 5) and with only 3 deducible grades (grades `2', `3',
and `4'), an increase in muscimol binding along the placental segment of the
Anthropoid ancestral lineage is probably present. There is no reason to think
that this would not continue into the human lineage.
Discussion
The primary results show that profiles of the relative density of muscimol
binding across the four major subdivisions of the thalamus are similar among
amniotic vertebrates regardless of their class, order or family. In contrast,
the results suggest that there are statistically reliable differences in
muscimol binding between mammals and non-mammals, between marsupial and
placental mammals, and depending on their degree of kinship with Anthropoids,
among the placental mammals in the sample.
The Neurochemical Plan of Amniote and Mammalian Thalamus
The constancy of the relatively dense muscimol binding in the dorsal
thalamus across the nine diverse species of amniotes suggests that the
presence of high affinity GABA-A receptors in the dorsal thalamus may be a
fundamental principal of neural organization at least among later vertebrates.
As noted above, similarity of particular receptor types across a variety of
species, orders, classes, and even phyla has been noted by others and has
obvious implications regarding neurochemical `conservation' over long periods
of brain evolution (eg., Cole et al., 1984, Mann and Enna 1980, Nielsen et
al., 1978). One is inclined to think of directional selection tending to alter
a species, rather than to keep it constant. In point of fact, stabilizing
selection is probably usually maintaining the constancy of a character rather
than altering it (i.e. see Sheppard, 1960). This seems to be true even at the
level of the biochemistry of the synapses. Second, the increase of muscimol
binding in mammals compared to non-mammals suggests an increase in
high-affinity GABA-A receptors in mammals and therefore, a greater role of
inhibitory processing in the mammalian variety of dorsal thalamus. Because
there are close parallels in the evolutionary development of dorsal thalamus
and cerebral neocortex, a difference in the thalamus in mammals (see
hypothetical ancestor `1' in Fig. 1) and their premammalian ancestors (`0' in
Fig. 1) might well be a concomitant of the enhanced cortical development of
the cerebrum seen in the mammals over the same period of geological time --
perhaps a demand placed on the dorsal thalamus by the emergence of mammalian
cortex itself.(e.g., Nauta and Karten 1970, Northcutt 1984, Pritz and Stritzel
1994, Rose and Woolsey 1949, Veenman et al., 1994, Butler, 1994). That may
mean for human evolution there has been a progressive increase not only in
cortex but also in the thalamus supporting the cortical development. Further,
along with selective pressure for an increase in cortical size has come an
increase in the inhibitory receptors and therefore the ability to inhibit
ongoing activity.
Finally, the notion that the GABAergic gating in the thalamus might have
been altered during the further evolution of the Primate (and therefore human)
brain is generally supported by the results of the present study (cf, Cole et
al.,1984, Mann and Enna 1980, Nielsen et al., 1978, Penny et al., 1984).
Since the dorsal thalamus eventually achieved a high density both of
GABA-A receptors and GABA-ergic neurons in Primates (including humans), the
dorsal thalamus seems to have had a unique and persistent status in its
potential for inhibitory and probably disinhibitory gating of information
directed toward the cerebral cortex. Perhaps this is the basis for the saying
that the mark of intelligence lies as much in what one does not say or do as
it does in one's words or actions!
Acknowledgements
I dedicate this manuscript to the late R. Bruce Masterton because it was
in his continued search for links with our common ancestry that the seeds of
this research were born. I also thank Bobbi Baker for her knowledge and skill
with receptor binding techniques. This work was supported in part by NIH grant
NS07726.
References
Ariens Kappers, C.U.
1929 The Evolution of the nervous system in invertebrates, Vertebrates and
man. Bohn, Haarlem.
Beaumont, K., Chilton, W. W., Yamamura, H. I. & Enna, S. J.
1978 Muscimol binding in rat brain: Association with synaptic GABA
receptors. Brain Res. 148: 153-162.
Butler, A.B.
1994 The evolution of the dorsal thalamus of jawed vertebrates, including
mammals: cladistic analysis and a new hypothesis. Brain Research Reviews 19:
29-65
Cole, L. M., Lawrence, L. J., & Casida, J. E.
1984 Similar properties of 35S-t-butylbicyclophosphorothionate receptor
and coupled components of the GABA receptor-ionophore complex in brains of
human, cow, rat, chicken and fish. Life Sci. 35: 1755- 1762.
Cronin, J. E. & Sarich, V. M.
1980 Tupaiid and archonta phylogeny: The macromolecular evidence. In
Luckett, W. P. Ed. Comparative Biology and Evolutionary Relationships of Tree
Shrews. New York: Plenum Press, pp. 293-312.
Dene H., Goodman, M., Prychodko, W. & Matsuda, G.
1980 Molecular evidence for the affinities of Tupaiidae In Luckett, W. P.
Ed. Comparative Biology and Evolutionary Relationships of Tree Shrews. New
York: Plenum Press, pp. 269-291.
Diamond, I.T., and Hall, W.C.
1969 Evolution of neocortex. Science 164: 251-262.
Geary W. A., Toga, A. W. & Wooten, G. F.
1985 Quantitative film autoradiography for tritium: Methodological
considerations. Brain Res. 336: 334-336.
Glendenning, K. K. & Baker, B. N.
1988 Neuroanatomical distribution of receptors for three potential
inhibitory neurotransmitters in the brainstem auditory nuclei of the cat. J.
Comp. Neurol. 275: 288-308.
Kuhar, M. J.
1985 Receptor localization with the microscope. In Yamamura, H. I., Enna,
S. J., & Kuhar, M. J. Eds. Neurotransmitter Receptor Binding, 2nd ed. New
York: Raven Press, pp. 153-176.
Luckett, W. P.
1980 The use of reproductive and developmental features in assessing
Tupaiid affinities. In Luckett, W. P. Ed. Comparative Biology and Evolutionary
Relationships of Tree Shrews. New York: Plenum Press pp. 245-266.
Mann, E. & Enna, S. J.
1980 Phylogenetic distribution of bicuculline-sensitive g-aminobutyric
acid (GABA) receptor binding. Brain Res. 184: 367-373.
McKenna M. C.
1975 Toward a phylogenetic classification of Mammalia. In Luckett, W. P. &
Szalay, F.S., Eds. Phylogeny of the Primates. New York: Plenum Press, pp.
21-43.
Nauta, W. J. H. & Karten, H. J.
1970 A general profile of the vertebrate brain with sidelights on the
ancestry of cerebral cortex. In Schmitt, F.O. Ed. The Neurosciences: Second
Study Program. New York: The Rockefeller University Press,. pp. 7-26.
Nielsen, M., Braestrup, C. & Squires, R. F.
1978 Evidence for a late evolutionary appearance of brain-specific
benzodiazepine receptors: An investigation of 18 vertebrate and 5 invertebrate
species. Brain Res. 141: 342-346.
Northcutt R. G.
1984 Evolution of the vertebrate central nervous system: Patterns and
processes. Amer. Zool. 24: 701-716.
Novacek M. J.
1992 Mammalian phylogeny: Shaking the tree. Nature 356: 121-125.
Nudo R. J. and Masterton R. B.
1998 Descending pathways to the spinal cord: A comparative study of 22
mammals. J. Comp. Neurol. 277: 53-79.
Pazos A. and Palacios J. M.
1985 Quantitative autoradiographic mapping of serotonin receptors in the
rat brain. I. Serotonin-L receptors. Brain Res. 346: 205-230.
Penny, G. R., Conley, M., Schmechel, D. E. & Diamond, I. T.
1984 The distribution of glutamic acid decarboxylase immunoreactivity in
the diencephalon of the opossum and rabbit. J. Comp. Neurol. 228: 38-56.
Penny, G. R., Fitzpatrick, D., Schmechel, D. E., & Diamond,I.T.
1983 Glutamic acid decarboxylase-immunoreactive neurons and horseradish
peroxidase-labeled projection neurons in the ventral posterior nucleus of the
cat and Galago senegalensis. J. Neurosci. 3: 1868-1887.
Penney, J. B., Pan, H. S., Young, A. B., Frey, K. A. & Dauth, G. W.
1981 Quantitative autoradiography of 3H-muscimol binding in rat brain.
Science 214: 1036-1038.
Polyak, S
1957 The Vertebrate Visual System (H. Kluver, ed.). Univ. of Chicago
Press, Chicago, Illinois.
Pritz, M. B. & Stritzel, M. E.
1994 Glutamic acid decarboxylase immunoreactivity in some dorsal thalamic
nuclei in Crocodilia. Neurosci. Lett. 165: 109-112.
Ramon y Cajal, S.
1909 Histologie du Systeme Nerveux de l'Homme et des Vertebres, 2 Vols.
Maloine, Paris.
Ramon y Cajal, S.
1966 Studies on the Diencephalon (transl. Romon Moliner E). Charles C.
Thomas, Springfield, IL.
Report of the AVMA Panel on Euthanasia
1993 JAVMA 202: 230-249.
Rose, J. E. & Woolsey, C. N.
1949 Organization of the mammalian thalamus and its relationships to the
cerebral cortex. Electroencephalogr. Clin. Neurophysiol. 1: 391-403.
Shaw, C., Needler, M. C. & Cynader, M.
1984 Ontogenesis of muscarinic acetylcholine binding sites in cat visual
cortex: Reversal of specific laminar distribution during the critical period.
Dev. Brain Res. 14: 295-299.
Sheppard, P.M.
1960 Natural Selection and Heredity. Harper and Row, New York.
Snodgrass, S. R.
1978 Use of 3H-muscimol for GABA receptor studies. Nature 273:392-394.
Yamamura, H. I. & Snyder, S. H.
1974 Muscarinic cholinergic binding in rat brain. Proc. Natl. Acad. Sci.
USA 71: 1725-1729.
Zarbin, M. A., Wamsley, J. K. & Kuhar, M. J.
1981 Glycine receptor: Light microscopic autoradiographic localization
with [3H] strychnine. J. Neurosci. 1: 532-547.
Table Caption
TABLE I. Specific muscimol binding (total binding minus nonspecific
binding) in the four subdivisions and the total diencephalon for the seven
mammals and two non-mammals. In each case the greatest degree of binding was
found in the dorsal thalamus.
Figure Legends
Figure 1. Relationship of the nine amniotes in the present sample with
five successive hypothetical ancestors in the Anthropoid lineage. Premammalian
(0), mammalian (1), placental (2A,2B), archontan (3; Luckett, 1980), and
primate (4) ancestral grades are arbitrarily labeled to yield a total of 5
distinguishable grades. Note that if edentates (2A; Armadillos) are accepted
as having more remote common ancestry with Anthropoids than rodents (2B; e.g.,
Novacek 1992) then the `last common ancestor' scale would expand to a total of
6 separable grades, with 4 grades within placental mammals alone.
Figure 2. Four scatterplots of the distribution of bound muscimol in
dorsal thalamus (left) or entire diencephalon (right). Top graphs compare
non-mammals and mammals. The difference between non-mammals and mammals is
statistically reliable only for dorsal thalamus (see gray p-value in top
left). Bottom graphs compare marsupials and placentals. The difference between
marsupials and placentals is statistically reliable both for the dorsal
thalamus (bottom, left) and for the entire diencephalon (bottom, right).
Figure 3. Muscimol specific-binding in the subdivisions of the
diencephalon for the seven mammals and two non-mammals relative to the binding
in dorsal thalamus. Note that the binding in the dorsal thalamus is the most
dense in each species while the binding in the hypothalamus is more dense than
in either the epithalamus or ventral thalamus.
Figure 4. Specific muscimol binding for the nine animals arranged on the
basis of recency of their last common ancestor with mankind. Three lines in
each graph show relationships of binding in successively smaller subsets:
amniotes (n=9), mammals (n=7), and the placental mammals (n=5).Only the
relationship between muscimol binding in the dorsal thalamus among the five
placental mammals is statistically reliable (see shaded label in the left
graph).
Transtopia
- Main
- Pierre Teilhard De Chardin
- Introduction
- Principles
- Symbolism
- FAQ
- Transhumanism
- Cryonics
- Island Project
- PC-Free Zone
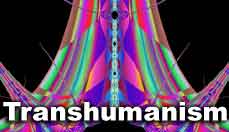
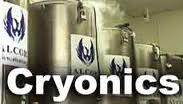
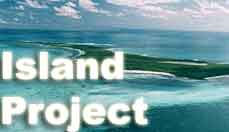
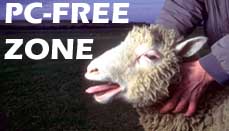
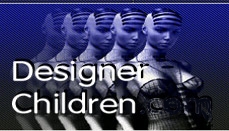
Prometheism News
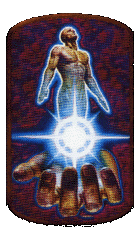